Piezoelectric biomaterials have attracted great attention owing to the recent recognition of the impact of piezoelectricity on biological systems and their potential applications in implantable sensors, actuators, and energy harvesters. However, their practical use is hindered by the weak piezoelectric effect caused by the random polarization of biomaterials and the challenges of large-scale alignment of domains.*
In the article “Active self-assembly of piezoelectric biomolecular films via synergistic nanoconfinement and in-situ poling” Zhuomin Zhang, Xuemu Li, Zehua Peng, Xiaodong Yan, Shiyuan Liu, Ying Hong, Yao Shan, Xiaote Xu, Lihan Jin, Bingren Liu, Xinyu Zhang, Yu Chai, Shujun Zhang, Alex K.-Y. Jen and Zhengbao Yang present an active self-assembly strategy to tailor piezoelectric biomaterial thin films.*
The nanoconfinement-induced homogeneous nucleation overcomes the interfacial dependency and allows the electric field applied in-situ to align crystal grains across the entire film. The β-glycine films exhibit an enhanced piezoelectric strain coefficient of 11.2 pm V−1 and an exceptional piezoelectric voltage coefficient of 252 × 10−3 Vm N−1. Of particular significance is that the nanoconfinement effect greatly improves the thermostability before melting (192 °C). *
This finding offers a generally applicable strategy for constructing high-performance large-sized piezoelectric bio-organic materials for biological and medical microdevices.*
The piezoelectric properties of the as-prepared β-glycine nanocrystalline films were evaluated by piezoresponse force microscopy (PFM) measurements.*
For all piezoresponse force microscopy (PFM) measurements and SKPM (scanning Kelvin probe force microscopy) measurements mentioned in this article, conductive NanoWorld Arrow-EFM AFM probes with PtIr coating on both AFM cantilever and AFM tip were used. The nominal resonance frequency and the nominal stiffness of the AFM probe are 75 kHz and 2.8 N m−1, respectively.
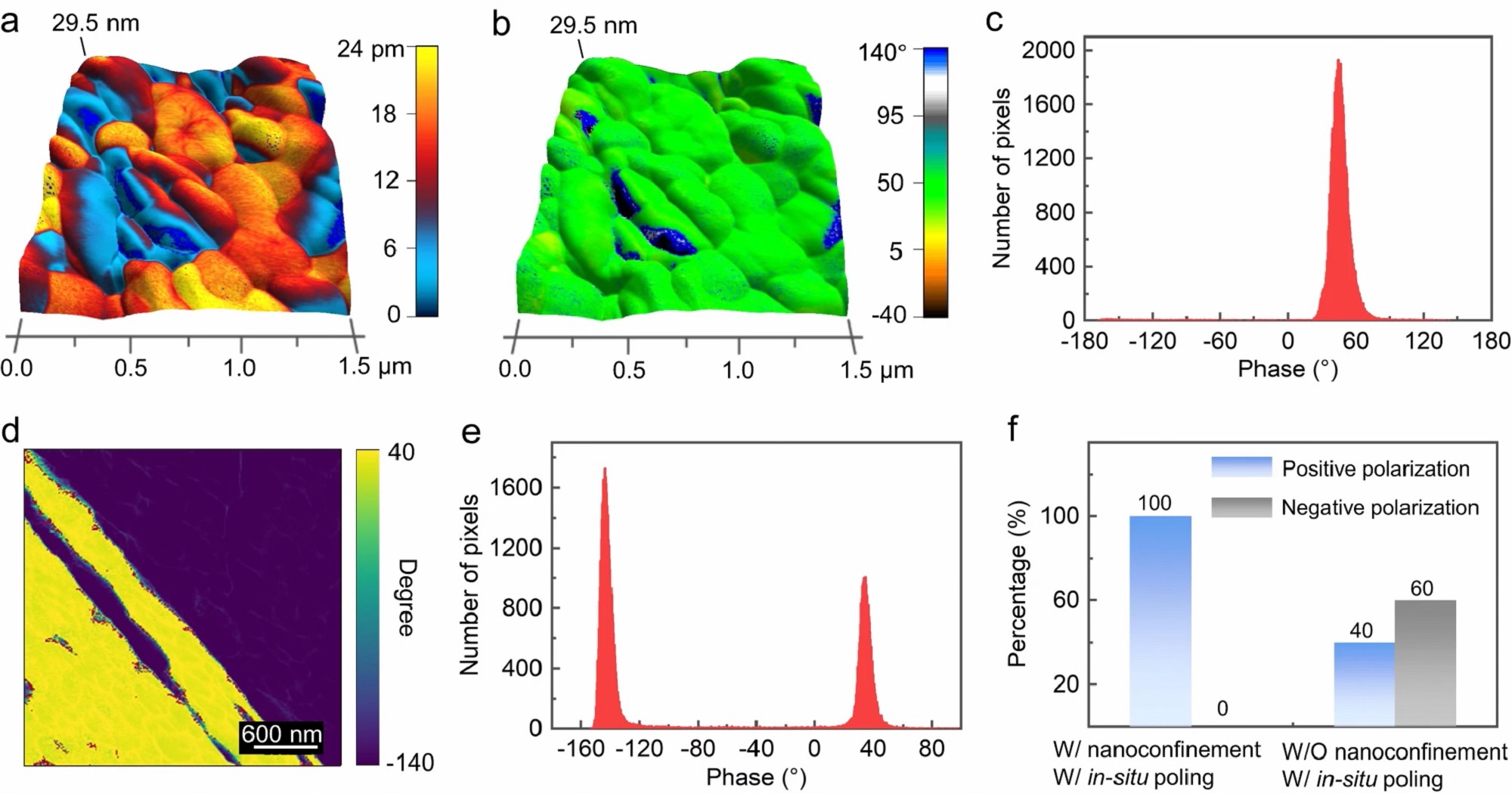
PFM measurements and polarization alignment studies of β-glycine nanocrystalline films.
a The PFM OOP amplitude mapping overlaid on the 3D topography of as-prepared films in a 1.5 × 1.5 µm2 area. The applied AC voltage is 2 V. b The corresponding PFM OOP phase mapping overlaid on the 3D topography. c Histogram calculated from the PFM OOP phase mapping in (b) showing that the β-glycine nanocrystalline films are dominated by domains with the unique polarization direction. d PFM OOP phase mapping of the β-glycine microcrystals obtained by electrohydrodynamic focusing deposition through heterogeneous nucleation. e Histogram calculated from the phase mapping in (d). f Comparison of statistics of the piezoelectric phase for the as-prepared β-glycine nanocrystalline films via synergistic nanoconfinement and in-situ poling (left), and β-glycine microcrystals grown by heterogeneous nucleation in the absence of nanoconfinement effect (right).
*Zhuomin Zhang, Xuemu Li, Zehua Peng, Xiaodong Yan, Shiyuan Liu, Ying Hong, Yao Shan, Xiaote Xu, Lihan Jin, Bingren Liu, Xinyu Zhang, Yu Chai, Shujun Zhang, Alex K.-Y. Jen and Zhengbao Yang
Active self-assembly of piezoelectric biomolecular films via synergistic nanoconfinement and in-situ poling
Nature Communications volume 14, Article number: 4094 (2023)
DOI: https://doi.org/10.1038/s41467-023-39692-y
Please follow this external link to read the full article: https://rdcu.be/dzddh
The article “Active self-assembly of piezoelectric biomolecular films via synergistic nanoconfinement and in-situ poling” by Zhuomin Zhang, Xuemu Li, Zehua Peng, Xiaodong Yan, Shiyuan Liu, Ying Hong, Yao Shan, Xiaote Xu, Lihan Jin, Bingren Liu, Xinyu Zhang, Yu Chai, Shujun Zhang, Alex K.-Y. Jen and Zhengbao Yang is licensed under a Creative Commons Attribution 4.0 International License, which permits use, sharing, adaptation, distribution and reproduction in any medium or format, as long as you give appropriate credit to the original author(s) and the source, provide a link to the Creative Commons license, and indicate if changes were made. The images or other third-party material in this article are included in the article’s Creative Commons license, unless indicated otherwise in a credit line to the material. If material is not included in the article’s Creative Commons license and your intended use is not permitted by statutory regulation or exceeds the permitted use, you will need to obtain permission directly from the copyright holder. To view a copy of this license, visit https://creativecommons.org/licenses/by/4.0/.