Extremely robust cohesion triggered by calcium silicate hydrate (C–S–H) precipitation during cement hardening makes concrete one of the most commonly used man-made materials. *
In the article “Detecting Early-Stage Cohesion Due to Calcium Silicate Hydration with Rheology and Surface Force Apparatus” Teresa Liberto, Andreas Nenning, Maurizio Bellotto, Maria Chiara Dalconi, Dominik Dworschak, Lukas Kalchgruber, Agathe Robisson, Markus Valtiner and Joanna Dziadkowiec present a proof-of-concept study, in which they seek an additional nanoscale understanding of early-stage cohesive forces acting between hydrating model tricalcium silicate (C3S) surfaces by combining rheological and surface force measurements. *
The composition and surface properties of the PLD-deposited calcium silicate films have been analyzed by X-ray diffraction (XRD), X-ray photoelectron spectroscopy (XPS), scanning electron microscopy with energy-dispersive spectroscopy (SEM-EDS), and atomic force microscopy (AFM). *
The calcium silicate surfaces were initially scanned in air. Subsequently, the authors injected about 1 mL of MilliQ water on top of the films so that both the sample and the AFM tip were submersed and followed the evolution of topography within the same region on a surface. The resultant images were processed in AR software by applying a 5 × 5 median filter. Roughness values were reported as root-mean-square (rms) values of the measured surface heights. *
Teresa Liberto et al. further used Atomic Force Microscopy AFM to study the nanoscale details of the film topography. The measurements performed in air revealed that the calcium silicate films are polycrystalline and are composed of uniform-sized nanograins, smaller than 100 nm in diameter (Figure 6A). At larger scan sizes, they also detected a significant amount of much larger, micron-sized particles that contribute to the quite high surface roughness; however, these were mostly located on sample edges, away from the PLD plume center.*
Subsequent AFM measurements in liquid confirmed that the films do not undergo full dissolution in water for several hours, as tested by continuously scanning the surface fully immersed in water as shown in Figure 6B. The rms roughness of the films in air was 1.2 nm (scan size 1 × 1 μm2), and it significantly increased upon exposure to H2O (rms up to 7 nm for a scan size of 1 × 1 μm2; see Figure 6C). *
The authors also detected a significant change in the film topography in water, with nanoparticles becoming less defined on a surface. This indicates that the films reprecipitated or swelled in contact with water, suggesting the gel-like character of the reprecipitated layer.*
However, despite the low thickness of the PLD-deposited films, there was no indication of complete dissolution–reprecipitation of the films: a smooth mica substrate topography that would indicate film dissolution was not exposed and a rough particle-laden surface was preserved throughout the whole measurement in water. In addition, there was no evidence of complete film dissolution in the SFA measurements; dissolution-related reduction in film thickness would have been indicated by the SFA-coupled white-light interferometric fringes. Therefore, the thin films behave as good model systems to study the early dissolution–reprecipitation phase by microscale surface force measurements. *
NanoWorld ARROW-UHFAuD AFM probes were used for the Atomic Force Microscopy.
The findings presented in the article confirm the strong cohesive properties of hydrated calcium silicate surfaces that, based on the authors’ preliminary SFA measurements, are attributed to sharp changes in the surface microstructure. In contact with water, the brittle and rough C3S surfaces with little contact area weather into soft, gel-like C–S–H nanoparticles with a much larger surface area available for forming direct contacts between interacting surfaces. *
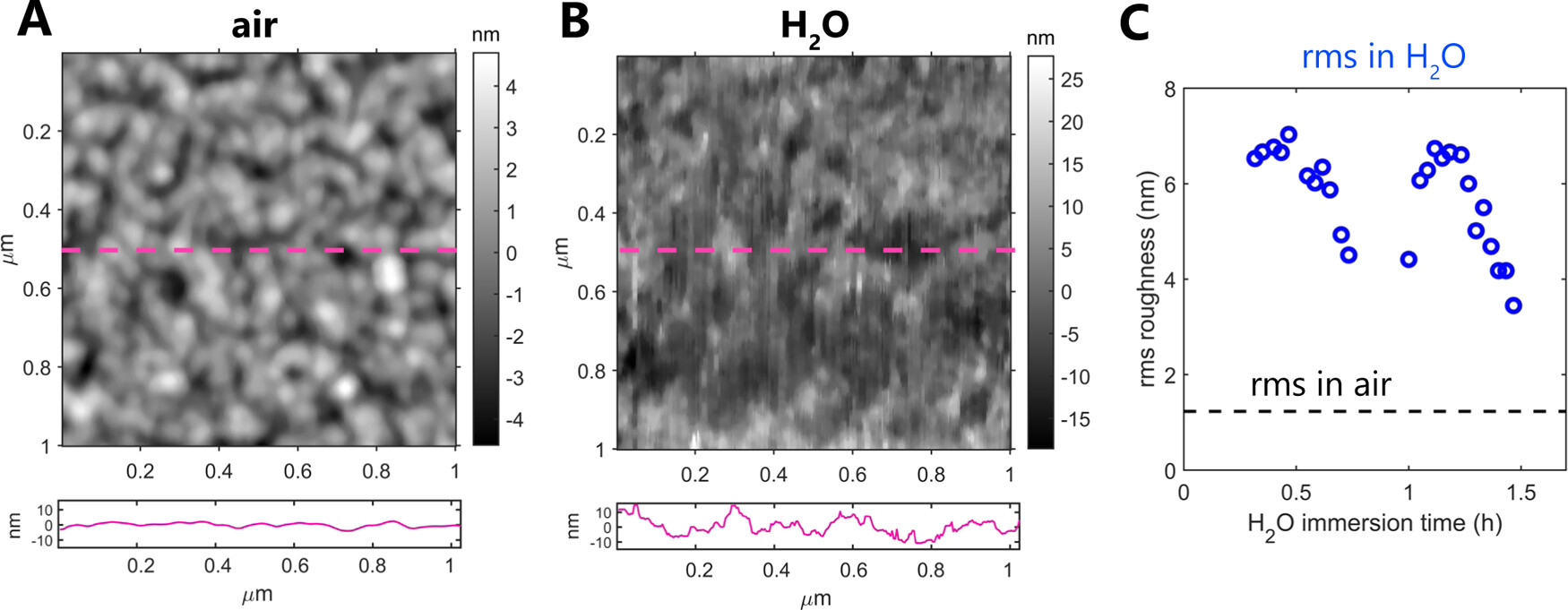
Atomic force microscopy topography maps of calcium silicate films in air (A) and in water ((B) sample immersed in H2O for 30 min). The panels below AFM maps show height profiles along the center of each AFM image as marked with a dashed magenta line. Note that the y axis is the same in both panels. (C) Comparison of the root-mean-square (rms) roughness measured in air and in water (over 1.5 h in the same position) for a 1 × 1 μm2 scan size. Each point corresponds to one AFM scan, including the measurement in air.
*Teresa Liberto, Andreas Nenning, Maurizio Bellotto, Maria Chiara Dalconi, Dominik Dworschak, Lukas Kalchgruber, Agathe Robisson, Markus Valtiner and Joanna Dziadkowiec
Detecting Early-Stage Cohesion Due to Calcium Silicate Hydration with Rheology and Surface Force Apparatus
Langmuir 2022, 38, 48, 14988–15000
DOI: https://doi.org/10.1021/acs.langmuir.2c02783
The article “Detecting Early-Stage Cohesion Due to Calcium Silicate Hydration with Rheology and Surface Force Apparatus” by Teresa Liberto, Andreas Nenning, Maurizio Bellotto, Maria Chiara Dalconi, Dominik Dworschak, Lukas Kalchgruber, Agathe Robisson, Markus Valtiner and Joanna Dziadkowiec is licensed under a Creative Commons Attribution 4.0 International License, which permits use, sharing, adaptation, distribution and reproduction in any medium or format, as long as you give appropriate credit to the original author(s) and the source, provide a link to the Creative Commons license, and indicate if changes were made. The images or other third-party material in this article are included in the article’s Creative Commons license, unless indicated otherwise in a credit line to the material. If material is not included in the article’s Creative Commons license and your intended use is not permitted by statutory regulation or exceeds the permitted use, you will need to obtain permission directly from the copyright holder. To view a copy of this license, visit https://creativecommons.org/licenses/by/4.0/.